Recently, a research team led by Han Mengdi from Peking University has developed a new material—hard magnetic conductive composite material. Compared to previous reconfigurable electronic devices, this flexible electronic device based on the hard magnetic conductive composite material has the following advantages:
Firstly, it is not only reconfigurable in function but also in sensitivity and spatial coverage. This is because the hard magnetic conductive composite material can serve both as a sensing material and as an electrical interface for flexible electronic devices.
Secondly, it does not rely on additional materials (such as liquid media for capillary force connections), nor does it depend on equipment such as laser machines or 3D printers. This is because, for the hard magnetic conductive composite material, it can achieve reversible self-alignment electrical connections with the assistance of magnetism.
At the same time, the synergistic effect of the porous graphene conductive network, magnetic particles, and magnetic domains can enhance the performance of flexible electronic devices based on the hard magnetic conductive composite material, improving their effectiveness in electrochemical sensing, electrophysiological sensing, and temperature sensing.
Advertisement
Specifically: for electrochemical sensing, magnetic particles can promote electron transfer, thereby improving sensing sensitivity; for electrophysiological sensing, due to the hydrophilicity of doped magnetic particles, the electrode impedance of electrophysiology will also be reduced; for temperature sensing, the internal electron thermal motion and magnetic domain thermal motion of magnetic particles can enhance the sensitivity to temperature.Thirdly, for flexible electronic devices based on hard magnetic conductive composite materials, due to their hard magnetic properties, they can achieve connections with other electronic components through reversible and self-aligning methods.
In this way, by adjusting the magnetization strength of the magnetic particles, or adjusting the proportion of doped magnetic particles, the interfacial adhesion between the two hard magnetic conductive composite materials can be regulated, allowing them to form electrical connections and achieve reversible assembly and disassembly.
Experiments on healthy subjects have shown that flexible electronic devices based on hard magnetic conductive composite materials can measure electrocardiogram signals, skin impedance, skin temperature, and the concentration of ions and metabolites in sweat, with reconfigurable characteristics in sensitivity, spatial distribution, and sensing modes.
It can be seen that hard magnetic conductive composite materials are a promising material that can be used to construct reconfigurable flexible electronic devices for continuous, multimodal, and customizable measurements of physical and biochemical signals, thus playing a role in various biomedical fields to assist in diagnosis and treatment.
In addition, the research team has also analyzed the sensing mechanism of this material - the synergistic effect of porous conductive networks, magnetic particles, and magnetic domains.Through this, an advanced material is provided for the preparation of flexible electronic devices, which helps to create reconfigurable flexible electronic devices that can be manually connected and manually separated, and provides potential opportunities for human-computer interfaces and wearable devices.
In general, reconfigurable flexible electronic devices based on magnetic conductive composite materials have a wide range of potential application value, especially in the following two aspects:
Firstly, the application of individual devices that can achieve reconfigurable performance.
In the application of numerous sensors, the contradiction between sensitivity and sensing range has always been a difficult problem to overcome. In the past, to improve the sensitivity of a sensor, it was often necessary to sacrifice its detection range, and vice versa.
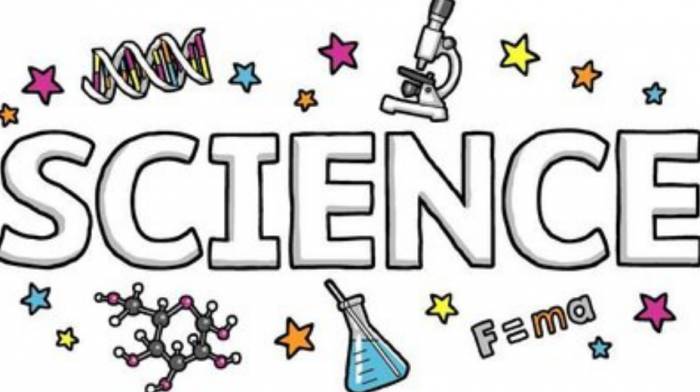
However, this team used the reversible magnetic attraction characteristics of magnetic materials to change the structure of the sensor, thereby achieving dynamic adjustment of sensing performance.This method can adjust the sensitivity and sensing range according to actual needs without replacing the sensor, thereby greatly improving the applicability and flexibility of the device.
For applications that require monitoring under different environmental conditions or different types of signals, the above capability is of great significance.
Secondly, it can achieve multimodal sensing applications.
In the field of biomedicine, the application of multimodal sensing technology is very critical. For specific diseases such as cancer and heart disease, which have complex causes and significant changes in the stages of disease development, traditional single-mode or fixed-mode sensors often cannot meet clinical needs.
The results of this study can achieve the on-demand reconstruction of sensor functions to adapt to the monitoring of different stages or different types of biological signals.For example, in the early stages of a disease, highly sensitive biochemical sensors may be required to detect trace amounts of biomarkers. In the treatment phase of the disease, parameters need to be adjusted to adapt to changes in the condition.
At the same time, this flexibility also has great potential in the fields of personalized medicine and real-time disease monitoring.
Overall, this reconfigurable technology based on magnetic conductive composite materials provides a new approach to solving the contradiction between the sensitivity and sensing range of traditional sensors, and also provides new possibilities for multimodal sensing and personalized monitoring. It can bring good application prospects in various fields such as intelligent manufacturing, environmental monitoring, and medical health.
It is reported that flexible electronic devices can continuously monitor a variety of bio-physical signals, such as heart rate, blood pressure, and body temperature, and can also monitor a variety of biochemical signals, such as ions and metabolites in body fluids, thus they can be used for continuous monitoring in biomedicine, as well as for operation and interaction in robots.
However, the sensitivity, functional modality, and spatial distribution of flexible electronic devices still cannot be adjusted on demand. This is mainly because the sensing layer and the electrical interface layer in the devices are completely fixed and cannot achieve reversible adjustment.In recent years, despite some achievements in reconfigurable flexible electronics, reconfiguration can only be carried out using specific materials or specific equipment, and thus lacks universality. Moreover, they can only perform one-dimensional reconfiguration, lacking the ability for multi-dimensional reconfigurability.
At the beginning of the research, Han Mengdi's team first surveyed the overall development trend of the flexible electronics field, and found that most flexible electronic devices have fixed functional structures and cannot be adjusted on demand.
The previously developed reconfigurable flexible electronic devices also had issues such as the necessity to rely on specific equipment or materials for reconfiguration, and the limitation to only one-dimensional reconfiguration. To address these issues, the team set this as their research topic.
Subsequently, they selected magnetic materials, conductive materials, and polymer materials, and comprehensively compared the flexibility, magnetism, conductivity, and robustness of these materials.
Ultimately, they decided to use neodymium iron boron as the magnetic material, polydimethylsiloxane as the polymer substrate, and laser-induced graphene as the conductive material.Subsequently, they continuously explored processing parameters and material ratios, adjusting the parameters for laser processing of conductive materials as well as the proportions of magnetic materials, conductive materials, and polymer substrates, thereby enabling the conductive structures to possess integrity, magnetic strength, and flexibility.
Furthermore, based on the magnetic conductive composite materials, they created various sensors and compared their performance.
Afterward, leveraging the magnetic assistance for electrical connection of the magnetic conductive composite materials, they designed through-hole structures to enhance the reversibility, robustness, and conductivity of the electrical interface. At the same time, they also designed self-alignment structures to improve alignment accuracy.
In addition, the research team also compared the effects of the magnetic assistance for electrical connection with the connection effects of commercially available conductive silver adhesive leads, verifying the reliability of the magnetic assistance for electrical connection.
Moreover, they characterized the flexibility of the electrical interface to ensure that wearable applications could be achieved.Finally, they utilized various sensors based on magnetic conductive composite materials, along with reversible self-alignment electrical connections, to construct multidimensional, reconfigurable flexible electronic devices. These devices were applied for health monitoring of subjects in various scenarios, specifically including reconfigurable applications of sensitivity, spatial distribution, and functional modalities.
Ultimately, the related paper was published in Advanced Materials with the title "Hard Magnetic Graphene Nanocomposite for Multimodal, Reconfigurable Soft Electronics" [1].
Peking University Ph.D. student Xiang Zehua is the first author, and Han Mengdi serves as the corresponding author.
In the future, they plan to comprehensively enhance and expand the reconfigurable flexible electronic technology based on hard magnetic conductive composite materials, which will cover the following aspects:
First, expand the sensing modalities.At present, the focus of this work is primarily on the electrochemical sensing module. Moving forward, they plan to shift their research emphasis to physical sensing modules, such as pressure and vibration, thereby enabling the sensor to monitor a more diverse range of physical and biochemical conditions, greatly enhancing the application field and practicality. At the same time, they will also delve into the impact of magnetic particles on the performance of different types of sensing modules.
Second, improve self-alignment interconnection technology.
In this regard, they plan to improve the current self-alignment interconnection technology to achieve more precise and programmable magnetic attraction control. This improvement will be particularly important for assembling small devices over a larger range, especially in the assembly at the micro-nano scale.
Through this method, more delicate and efficient device assembly can be achieved, providing the possibility for manufacturing smaller and more complex sensor systems.
Third, develop fully integrated reconfigurable flexible electronics.The long-term goal of the research group is to develop a fully integrated reconfigurable flexible electronic system, including the tight integration of front-end sensing modules and back-end circuit modules.
According to the needs of different application scenarios, the front-end modules and back-end circuit modules can be flexibly selected and reconfigured to achieve complete on-demand reconfigurability from devices to circuits. This allows the same system to be applicable to a variety of different application scenarios, thereby greatly improving adaptability and cost-effectiveness.
Fourth, the expansion and deepening of application scenarios.
With the development of the above technologies, they hope to apply this reconfigurable flexible electronic system to a wider range of fields, such as wearable devices, biomedical monitoring, environmental monitoring, intelligent manufacturing, etc.
Leave a Comment